Effect of Rock Cover on Small Mammal Abundance In a Montana Grassland
by
Abstract
We examined the influence of rock cover, as an indicator of presumable retreat site availability on the abundance of deer mice (Peromyscus maniculatus) and prevalence of Sin Nombre virus (SNV) using long-term live trapping and habitat data from three live trapping grids and a short- term (three month), spatially replicated study across three slopes in Cascade County, Montana. In our long-term study, we found that deer mice were more abundant at a live-trapping grid with greater rock cover, than two grids with less rock cover. There was a non-significant trend (P = 0.053) for deer mice to be more abundant in rocky sites in the short term study. In the long-term study, average SNV antibody prevalence among deer mice was slightly greater (5.0 vs. 3.5 % on average) at the live trapping grid with more rock cover, than the grid with less rock cover. We were unable to demonstrate differences in SNV antibody prevalence among treatments in the short-term study. Further studies are needed to elucidate the multiple determinants of deer mouse abundance and SNV prevalence in grassland ecosystem and other habitat types.
Introduction
The abundance of small mammals varies temporally and spatially among and within habitat types (e.g., Krebs, 1996). In many cases, however, the underlying determinants of variability in small mammal abundance are unknown. Variation in abundance can have consequences for pathogen transmission because host abundance may influence contact and transmission rates among individuals in a population (Keeling and Rohani 2008). For example, human incidence of nephropathia epidemica (caused by Puumala hantavirus) was related to abundance of the host Clethrionomys glareolus in Sweden (Niklasson et al., 1995). Studies that examine determinants of host abundance in nature, and the effects these factors may have on pathogen prevalence, are needed to help reduce human exposure.
Deer mice (Peromyscus maniculatus) are widespread omnivorous rodents, which occur in a variety of habitat types across North America (Kirkland and Layne 1989, Douglass et al. 2001). These rodents have been ideal organisms for studies of habitat relationships for many decades (e.g., Smith 1940, Douglass 1989, Matlack et al. 2001, Johnston and Anthony 2008) and in Montana their abundance varies among and within many habitat types (e.g., Douglass et al. 2001). Deer mouse abundance has been found to be positively related to shrub cover in a short grass prairie environment (Stapp and Van Horne 1997) throughout central and western Montana (Douglass 1989a, Douglass et al. 2001) and Western Colorado (Douglass 1989b). Furthermore, deer mice are reservoirs for Sin Nombre virus (SNV, Bunyaviridae:Hantavirus) (Nichol et al., 1993), a directly transmitted zoonotic pathogen that is transmissible to humans causing Hantavirus Pulmonary Syndrome (HPS), which has a high mortality rate (CDC, 2009). The abundance of deer mice is an important component of SNV transmission (e.g., Madhav et al. 2007) and human exposure risk (Childs et al. 1995). Both abundance of deer mice and SNV prevalence among mice can vary significantly over small spatial scales (Glass et al. 2000, Douglass et al. 2001). Therefore, studies that investigate variability in deer mouse abundance and how this is affected by habitat characteristics within an expansive habitat type are of value to understanding SNV transmission and human exposure risk.
In Montana deer mouse abundance and prevalence of antibodies to SNV are on average highest in sagebrush (Artemesia tridentata) dominated environments (Douglass et al. 2001), where sagebrush itself may provide retreat sites. However, deer mice can also be abundant, with high SNV antibody prevalence in other habitat types, such as grassland and forest environments (Kuenzi et al. 2001, Douglass et al., 2001). Here, we investigated how abundance of deer mice and prevalence of SNV antibodies varied within a grassland ecosystem located in Cascade County, Montana. We focused on rock cover, which may be an indicator of potential retreat site availability, as a determinant of variation in deer mouse abundance in this habitat type. Within this grassland several habitat characteristics were different among sampling locations and some, e.g., such as shrubs or patches of tall grass, may provide retreat sites for deer mice. However, we chose to focus on rock cover in this study because the abundance of deer mice at three long-term grassland live trapping grids differed (Douglass et al. 2001) as did rock cover. In addition, we frequently observed deer mice using rocks as retreat sites upon release from live traps. Although rock cover is clearly not critical to deer mouse survival in all habitats especially in open environments with high shrub cover (Douglass et al. 2001, Douglass 1989a, Douglass 1989b, Douglass and Frisina 1993), in this environment rock cover may be important for avoiding large predators, caching resources, or nesting. Other habitat characteristics differed among our long-term live trapping grids (see results) and likely also contributed to variation in deer mouse abundance within this grassland habitat type (Wecker 1963, Douglass 1989a, Douglass 1989b, Morris, 1997). The multivariate effect of habitat characteristics on deer mouse abundance will be the subject of further investigations.
We hypothesized that in a specific type of Northern Great Plain grassland, deer mice are more abundant, with a greater number of individuals and prevalence of SNV antibodies in the population, in sites with similar vegetative characteristics but greater rock cover (potential retreat site availability) than sites with less rock cover. We investigated the relationship between population abundance and prevalence of SNV in relation to rock cover in two ways. We used a long-term (1994-2010) study on population dynamics of deer mice on three live trapping grids and prevalence of SNV on two live trapping grids (Douglass et al. 1996, Douglass et al. 2001) from which our observations led to the hypothesis above. Because the long-term study lacked spatial replication in rock cover, we also undertook a short-term (three month) spatially- replicated study to evaluate the effect of rock cover on small mammal abundance.
Materials and Methods
Long-term data collection
Data on deer mice and habitat characteristics were collected on three one hectare grids (grid numbers 10, 11 and 12) located near Cascade (46° 59.3 N, 111° 35.3 W, 1408 m AMS), Montana. Grids were situated in grassland habitat supporting an active cattle ranch (Douglass et al., 1996). We live-trapped deer mice for three consecutive nights/month on all three 1-ha grids for 174 consecutive months between June 1994 and November 2008. Trapping grids consisted of 100 equally-spaced Sherman live traps (H. B. Sherman Traps. Tallahassee, Florida), baited with rolled oats and peanut butter and provisioned with polyester Fiberfil bedding. Upon capture, each rodent was given a uniquely numbered ear-tag (model #1005-1, National Band and Tag Co., Newport, KY) and species, gender, body mass, reproductive condition, and presence of scars or wounds were recorded. We routinely collected blood samples, which were later tested for antibodies to SNV, from grids 11 and 12 only. We followed animal handling, blood collection and safety precautions described by Mills et al. (1995) and approved by the University of Montana IACUC. Serological testing was performed at the Montana State Public Health Laboratory and at Special Pathogens Branch, U.S. Centers for Disease Control and Prevention, using methods described by Feldmann et al. (1993).
We used the enumeration technique of Chitty and Phipps (1966) to provide a minimum number of individuals known to be alive (MNA) during a 3-day trapping session as an index of population abundance for each month. The minimum number of deer mice antibody-positive to SNV (MNI) during each trap session was calculated in the same way for grids 11 and 12. Estimated antibody prevalence (EAP) of deer mice for a given month was calculated by dividing MNI by MNA (Mills et al. 1999a).
Data on habitat characteristics of the three long-term grids were collected twice annually (Jun and Sep) at 25 randomly- assigned locations/grid using a 10-pin point frame. Percent cover of lichens mosses, grass, forbs, shrubs, rock, leaf litter and bare ground was determined at each location. A contact with rock and a point frame rod was counted as rock cover. Although, direct use of rocks as retreat sites by deer mice was not quantified in this study, our assumption of rocks offering retreat sites seemed reasonable. Although our analysis did not directly account for use of rocks as retreat sites we found (1) large rocks that mice were able to move beneath were present at all sites, (2) small burrows were common around rocks at our sites, (3) mice were frequently observed retreating to rocks for cover when released from traps, and (4) mouse tracks and burrows in snow commonly originated from rocks.
Short-term Data Collection
To determine if abundance of deer mice differed among sites with more or less rock cover (retreat sites), we established six 0.25-ha grids on three slopes (named Hill One, Hill Two and Hill Three for this study) of approximately the same elevation and aspect. Within each slope, one grid was located in a rocky area and one in a non-rocky area. Grids were live-trapped for small mammals monthly from August-October 2008 in the same manner as the long-term live trapping grids, but with 25 equally spaced rather than 100 Sherman live-capture traps. Small mammal handling, testing for SNV antibodies, calculation of MNA, MNI rock cover, and SNV prevalence among deer mice was carried out in the same manner as the long-term trapping grids. In September, we also measured habitat characteristics at each of the six grids in the same way as the long-term grids, but with habitat characteristics determined from six randomly-assigned locations at each grid (a similar proportion/unit area as the long-term grids).
Analyses
We employed a Friedman analysis (Zar, 1996) to determine if abundance of deer mice, number of deer mice antibody positive for SNV, and antibody prevalence among deer mice differed among long- term trapping grids. A Friedman analysis was also used to determine if habitat characteristics differed among the long-term grids over time. Friedman analysis enabled examination of direction of differences among variables for individual sampling occasions collectively and, accordingly, was independent of the effects of seasonal and climatic forcing on dynamics, which are certainly important but beyond the scope of this investigation. For the short- term study, habitat characteristics among grids were compared using a Generalized Linear Model (GZLM), with hillside as a random effect. Optimal distribution and transformation of data (Poisson or negative binomial probability distribution and identity or log-link function respectively) for the GZLM were specified using an Omnibus test. A linear mixed model (LMM) was used to determine if deer mouse abundance and antibodies to SNV among deer mice (number antibody positive and antibody prevalence) differed between rocky and non-rocky sites, with month as a repeated measure and hillside as a random effect. All analyses were performed using SPSS 15.0 (SPSS Inc. Chicago, U.S.).
Results
Over the 15-yr long-term study, we captured 4458 individual deer mice 12,265 times in 155,700 trap-nights of effort. Abundance of deer mice fluctuated among months and years. Average monthly abundance of deer mice was greatest at grid 11 (MNA = 25.8), least at grid 12 (MNA= 15.3), and intermediate at grid 10 (MNA= 22.9) (Table 1). The average monthly number of antibody positive deer mice (MNI) and estimated antibody prevalence (EAP) was greater at grid 11 (MNI = 1.3, EAP = 0.05) than grid 12 (MNI = 0.3, EAP 0.035) (Table 1). However, on several occasions during 15 yrs of sampling, both grids 11 and 12 experienced many months with no presence of antibody-positive deer mice (Luis et al. 2010). Among the three long-term live trapping grids we detected significant differences in each of the habitat variables except bare ground (Table 2). Of particular focus in this study, rock cover was greatest at grid 11 (0.556 %) and least at grid 12 (0.147 %; Table 2).
We initially confirmed that the proportion of cover that consisted of rock was greater among sites we had selected as rocky sites, than non-rocky sites, in the short-term study (Table 3). Cover of most other habitat variables did not differ, except cover of lichens, which were found exclusively on rocks (Table 3). Over the 3-mo short-term study, 172 individual deer mice were captured 381 times in 1350 trap- nights. Deer mouse abundance was greatest at Hills One and Two and least abundant at Hill Three (Table 4). Average deer mouse abundance was higher at rocky sites than non-rocky sites but this difference was not statistically significant (Tables 4 and 5). The number of deer mice antibody positive for SNV and deer mouse antibody prevalence was unrelated to rocky or non-rocky habitat (Tables 4 and 5).
Discussion
Determining factors that influence zoonotic host abundance is important to understanding pathogen transmission, and subsequently human exposure risk. Here, we investigated if abundance of deer mice (a host reservoir for SNV) in a grassland
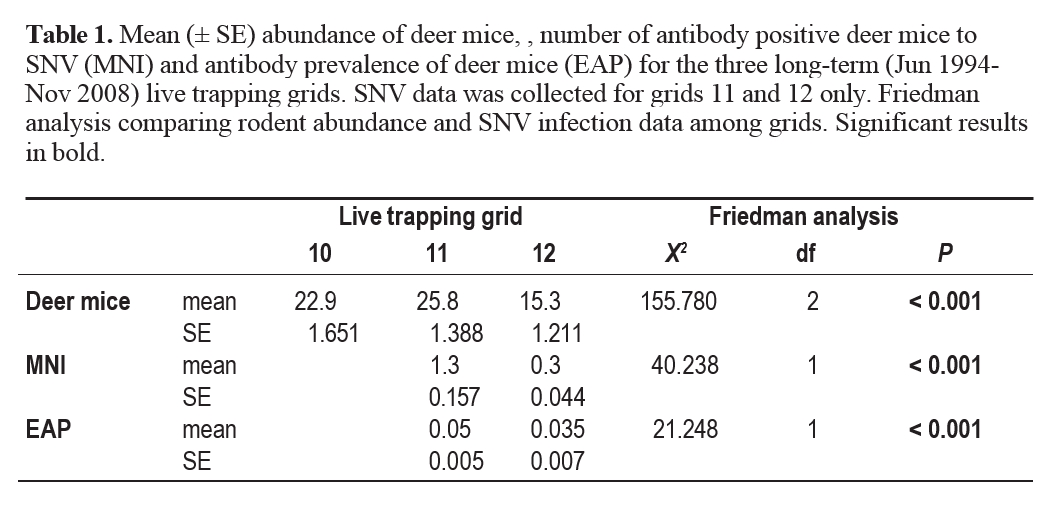
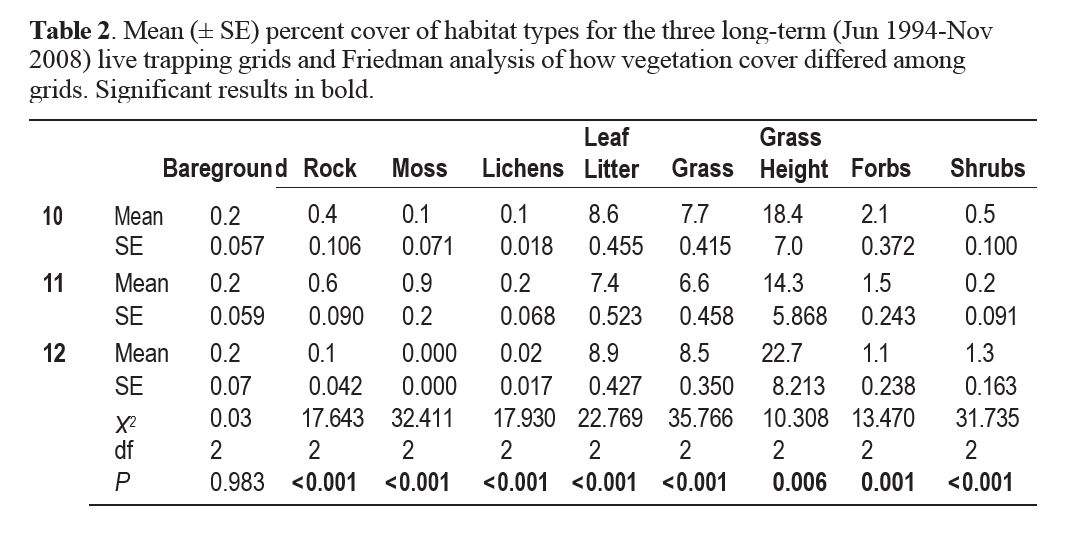
ecosystem was related to availability of rock cover, i.e., as an indicator of potential refuge site availability, and if prevalence of SNV antibodies was positively related to deer mouse abundance between rocky and less rocky areas. Using long-term data from three live trapping grids, we found deer mice more abundant at a site with more rock cover, than sites with less rock cover over many years. Deer mice were on average more abundant at rocky sites in the short-term study, but this was a non-significant trend (P = 0.053). Based on the long-term study, we also found the number and prevalence of SNV antibody positive deer mice was greater at sites with more rock cover, than sites with less rock cover, which supported our hypothesis. Our short-term study did not detect a significant difference in SNV between sites with more or less rock cover, which could have been due to the short duration and relatively low number of deer mice trapped.
Habitat characteristics varied among the three long-term grids in this study. This variation in habitat composition, in addition to rock cover, included moss, lichens, grasses, forbs, shrubs, and leaf litter. Among the three long-term grids, more deer mice were captured where cover of rocks, moss and lichens were high, and fewer were captured where cover of leaf litter, grasses and shrubs were high, suggesting these variables could all be determinants of deer mouse abundance in this grassland ecosystem. In previous work (Douglass 1989b) found a negative correlation between deer mouse abundance and grass cover. Douglass et al. (2001) also reported deer mice to be more abundant in sagebrush habitats in Montana. Shrubs in the current study area were mostly snowberry (Symphoricarpus spp.) with a growth form quite different from (particularly density of stems over the ground) sage. Collectively, our study, Douglass (1989b), and Douglass et al. (2001) indicated that deer mice are captured in less complex habitat matrices. We focused on rock cover as a source of variation in deer mouse abundance because of rock cover's potential use as retreat sites by deer mice. While releasing deer mice after capture we often observed them seeking refuge under rocks. In this grassland environment other types of retreat sites (such as logs and thick shrub cover) are absent or rare.
The habitat composition in our short- term study only differed by rock cover and lichens (which were observed on rocks only) among rocky and non-rocky grids. However, deer mice were generally but not statistically significantly so, more abundant at rocky grids. Our long-term study demonstrated that the number of deer mice with SNV antibodies and SNV antibody prevalence was higher on the grid with greater deer mouse abundance. This lends support to our hypothesis linking rock cover, host
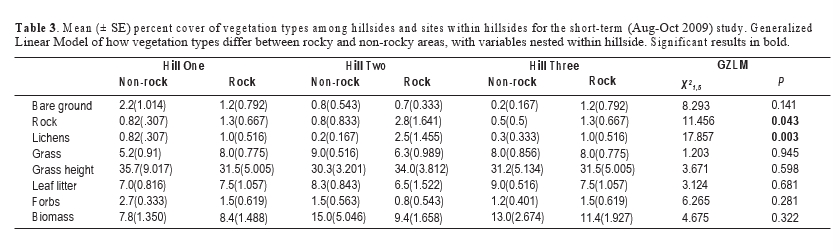
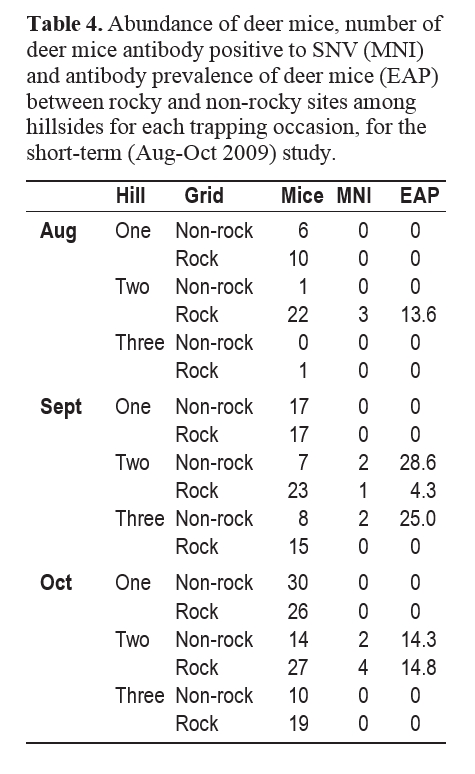
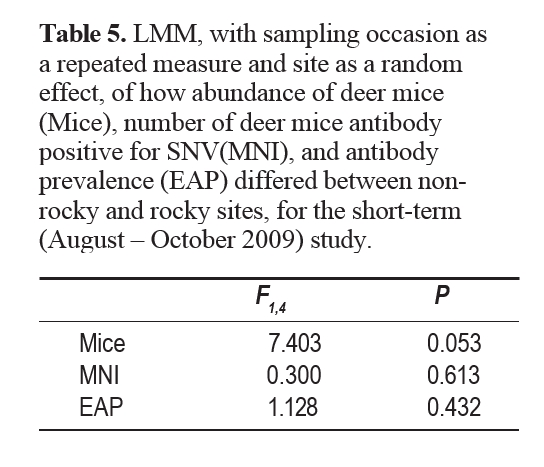
abundance and pathogen abundance in this grassland ecosystem. This relationship suggests that SNV prevalence is positively related to deer mouse abundance, which is consistent with some studies (e.g., Calisher et al. 1999, Yates et al. 2002, Carver et al. 2011a, and Madhav et al. 2007 and Carver et al. 2011b in a delayed density fashion).
Transmission of SNV is horizontal due to intraspecific interactions among deer mice, such as aggressive encounters (Mills et al. 1997, Root et al. 1999, Botten et al. 2002). Intraspecific interactions among deer mice may increase with increasing abundance, resulting in increased transmission events.
Our short-term study did not detect a relationship of deer mouse abundance with the number of SNV antibody positive individuals. Our short-term grids were only 0.25-ha in size (consisting of 25 Sherman live traps each), which limited the number of deer mice that could be trapped compared to the numbers captured on the larger long- term grids. Given the size of trapping grids, the lack of detectable differences in SNV at rocky and non-rocky grids may be due to study design. Conducting this short-term, spatially replicated study for a longer period of time and with one hectare trapping grids may enable better detection of relationships between 1-ha rock cover, deer mouse abundance and SNV among deer mice across this grassland landscape.
Other habitat characteristics can also function as sources of retreat sites to deer mice, particularly in other habitat types, leading to variation in abundance and infection. Douglass et al. (2001) found deer mouse abundance and SNV prevalence was greater in sagebrush habitat (where deer mice may use sagebrush as retreat sites) than grassland and forest habitats in western Montana. Root et al. (1999) found abundance of deer mice and the number of SNV positive individuals to be greater at a site dominated with sagebrush/juniper/pine than a site with oak/mixed grass /forbs in western Colorado. Lehmer et al. (2008), in a brief study, found deer mice were on average more abundant and with higher SNV prevalence at sites with less mechanical (off road vehicle) anthropogenic disturbance in the Great Basin Desert, Utah. However, in general, deer mice have been found to increase in numbers when habitats were opened by grazing (Smith 1940, Douglass and Frisina 1993, Matlack et al. 2001, Johnson and Anthony 2008) and forest treatments (Sullivan 1979, Douglass et al. 1999).
Our informal observations indicate that deer mice use rocks as retreat sites throughout the year. However, variation in deer mouse abundance across this landscape is likely related to a complex combination of habitat, climatic, and density dependant variables (i.e., Luis et al. 2010). It is also possible that at certain times of the year predators, such as weasels and snakes during summer months, may influence use of rocks by deer mice. Future spatially replicated analyses of other significant relationships among habitat characteristics and deer mouse abundance, particularly using multiple long-term studies, would be valuable to delineate all the underlying habitat determinants in this grassland ecosystem landscape. Studies evaluating interactions among retreat site use, other fauna and climatic factors would also be valuable. Telemetry studies (Douglass 1989a) and food habit studies (Van Horne 1982) would be valuable approaches to accurately determine how deer mice use this grassland habitat.
Acknowledgments
We thank the private ranch owner at Cascade for allowing us access to his property. Numerous individuals provided valuable assistance in the field including K. Coffin, R. Van Horn, C. Rognli, T. Wilson, W. Semmens, K. Hughes, A. Skypala, D. Waltee, B. Lonner, J. Wilson, A. Leary, J. Bertoglio, A. Alvarado, J. Trueax,C. Richardson and F. Arneson. K, Wagner provided database support, encouragement and general advice. S. Zantos provided valuable laboratory assistance. Financial support was provided by NIH grant P20 RR16455-06-07,08 from the INBRE- BRIN program of the National Center for Research Resources and the U.S. Centers for Disease Control and Prevention, Atlanta, GA, through cooperative agreement. K. Richardson was additionally supported by a grant from the Montana Tech undergraduate Research Program. This work followed all relevant environmental and institutional regulations in the collection of data presented here. The findings and conclusions presented here are those of the authors and do not necessarily represent the views of the funding agencies.
Literature Cited
Botten, J., K. Mirowsky, C. Y. Ye, K. Gottlieb, M. Saavedra, L. Ponce, and B. Hjelle. 2002. Shedding and intracage transmission of Sin Nombre hantavirus in the deer mouse (Peromyscus maniculatus) model. Journal of Virology 76:7587-7594.
Calisher, C. H., W. Sweeney, J. N. Mills, and B. J. Beaty. 1999. Natural history of Sin Nombre virus in western Colorado. Emerging Infectious Diseases 5:126-134.
Carver, S., J. N. Mills, A. Kuenzi, T. Flieststra, and R. Douglass. 2010. Sampling frequency differentially influences interpretation of zoonotic pathogen and host dynamics: Sin Nombre virus and deer mice. Vector-Borne and Zoonotic Diseases 10:575-583.
Carver, S., A. Kuenzi, K. H. Bagamian, J. N. Mills, P. E. Rollin, S. N. Zanto, and R. Douglass. 2011a. A temporal dilution effect: hantavirus infection in deer mice and the intermittent presence of voles in Montana. Oecologia. Ahead of Print. DOI 10.1007/s00442-00010-01882-z.
Carver, S., T. J. Trueax, R. Douglass, and A. Kuenzi. 2011b. Delayed density- dependent prevalence of Sin Nombre virus infection in deer mice (Peromyscus maniculatus) in central and western Montana. Journal of Wildlife Diseases 47:56-63
CDC. 2009. All about hantaviruses. Available: http://www.cdc.gov/ncidod/ diseases/hanta/hps/noframes/epislides/ episl5.htm. [Accessed Aug 18, 2009].
Childs, J. E., J. W. Krebs, T. G. Ksiazek, G. O. Maupin, K. L. Gage, P. E. Rollin, P. S. Zeitz, J. Sarisky, R. E. Enscore, J. C. Butler, J. E. Cheek, G. E. Glass, and C. J. Peters. 1995. A household-based, case- control study of environmental-factors associated with hantavirus pulmonary syndrome in the southwestern United States. American Journal of Tropical Medicine and Hygiene 52: 393-397.
Chitty, D, and E. Phipps. Seasonal changes in survival in mixed populations of two species of vole. Journal of Animal Ecology 35:313-331.
Douglass, R. J., 1989a. An Evaluation of Trap-revealed microhabitat selection; Using Radio-telemetry to test critical assumptions. Journal of Mammalogy 70:648-652
Douglass, R.J. 1989b. Assessment of the use of selected rodents in ecological monitoring. Environmental. Management 13:155-363.
Douglass, R. J. and M. R. Frisina. 1993. Mice and management on the Mount Haggin Wildlife Management Area. Rangelands 15:8-12.
Douglass, R.J., J. Quinn, K.W. Coffin, and G. Mariani. 1999. Initial effects of a landscape ecology treatment of a coniferous forest on small mammals. Intermountain Journal of Sciences 5:12-22.
Douglass, R. J., R. VanHorn, K. W. Coffin, and S. N. Zanto. 1996. Hantavirus in Montana deer mouse populations: preliminary results. Journal of Wildlife Diseases 32:527-530.
Douglass, R. J., T. Wilson, W. J. Semmens, S. N. Zanto, C. W. Bond, R. C. Van Horn, and J. N. Mills. 2001. Longitudinal studies of Sin Nombre virus in deer mouse-dominated ecosystems of Montana. American Journal of Tropical Medicine and Hygiene 65:33-41.
Feldmann H., A. Sanchez, S. Morzunov, C. F. Spiropoulou, P. E. Rollin, T. G. Ksiazek, C. J. Peters and S. T. Nichol. 1993. Utilization of autopsy RNA for the synthesis of the nucleocapsid antigen of a newly recognized virus associated with hantavirus pulmonary syndrome. Virus Research 30:351-367.
Glass, G. E., J. E. Cheek, J. A. Patz, T. M. Shields, T. J. Doyle, D. A. Thoroughman, D. K. Hunt, R. E. Enscore, K. L. Gage, C. Irland, C. J. Peters, and R. Bryan. 2000. Using remotely sensed data to identify areas at risk for hantavirus pulmonary syndrome. Emerging Infectious Diseases 6:238-247.
Johnston, A.N. and R.G. Anthony. 2008. Small-mammal microhabitat associations and response to grazing in Oregon. Journal of Wildlife Management 72:1736-1746.
Keeling, M. J. and P. Rohani. 2008. Modeling infectious diseases in humans and animals. Princeton University Press, Princeton.
Kirkland, G. L. and J. N. Layne. 1989. Advances in the study of Peromyscus (Rodentia). Texas Tech University Press, Lubbock.
Krebs, C. J. 1996. Population cycles revisited. Journal of Mammalogy 77:8-24.
Lehmer, E. M., C. A. Clay, J. Pearce-Duvet, S. S. Jeor, and M. D. Dearing. 2008. Differential regulation of pathogens: the role of habitat disturbance in predicting prevalence of Sin Nombre virus. Oecologia 155:429-439.
Luis, A. D., R. J. Douglass, J. N. Mills and O. N. Bjørnstad. 2010. The effect of seasonality, density and climate on the population dynamics of Montana deer mice, important reservoir hosts for Sin Nombre hantavirus. Journal of Animal Ecology 79:462-470.
Madhav, N. K., K. D. Wagoner, R. J. Douglass, and J. N. Mills. 2007. Delayed density-dependent prevalence of Sin Nombre virus antibody in Montana deer mice (Peromyscus maniculatus) and implications for human disease risk. Vector-Borne and Zoonotic Diseases 7:353-364.
Matlack, R. S., D. W. Kaufman, and G. A. Kaufman. 2001. Influence of grazing by bison and cattle on deer mice in burned tall grass prairie. American Midland Naturalist 146:361-368.
Mills, J. N., T. G. Ksiazek, B. A. Ellis, P. E. Rollin, S. T. Nichol, T. L. Yates, W. L. Gannon, C. E. Levy, D. M. Engelthaler, T. Davis, D. T. Tanda, J. W. Frampton, C. R. Nichols, C. J. Peters, and J. E. Childs. 1997. Patterns of association with host and habitat: Antibody reactive with Sin Nombre virus in small mammals in the major biotic communities of the southwestern United States. American Journal of Tropical Medicine and Hygiene 56: 273-284.
Morris, D. W. 1997. Optimally foraging deer mice in prairie mosaics: a test of habitat theory and absence of landscape effects. Oikos 80: 31-42.
Nichol, S. T., C. F. Spiropoulou, S. Morzunov, P. E. Rollin, T. G. Ksiazek, H. Feldmann, A. Sanchez, J. Childs, S. Zaki, and C. J. Peters. 1993. Genetic identification of a hantavirus associated with an outbreak of acute respiratory illness. Science 262: 914-917.
Niklasson, B., B. Hornfeldt, A. Lundkvist, S. Bjorsten, and J. Leduc. 1995. Temporal dynamics of Puumala virus antibody prevalence in voles and of nephropathia epidemica incidence in humans. American Journal of Tropical Medicine and Hygiene 53:134-140.
Root, J. J., C. H. Calisher, and B. J. Beaty. 1999. Relationships of deer mouse movement, vegetative structure, and prevalence of infection with Sin Nombre virus. Journal of Wildlife Diseases 35:311-318.
Smith, C.C. 1940. The effect of overgrazing and erosion upon the biota of the mixed- grass prairie of Oklahoma. Ecology 21:381-397.
Stapp, P. and B. Van Horne. 1997. Response of deer mice (Peromyscus maniculatus) to shrubs in shortgrass prairie: linking small-scale movements and the spatial distribution of individuals. Functional Ecology 11:644-651.
Sullivan, T.P. 1979. Clear-cut habitat and conifer seed predation by deer mice. Journal of Wildlife Management 43:861-871.
Van Horne, B. 1982. Niches of Adult and juvenile deer mice (Peromyscus maniculatus) in seral stages of coniferous forest. Ecology 63:992-1003.
Wecker, S. C. 1963. The role of early experience in habitat selection by the prairie deer mouse, Peromyscus maniculatus bairdi. Ecological Monographs 33:307-325.
Yates, T. L., J. N. Mills, C. A. Parmenter, T. G. Ksiazek, R. R. Parmenter, J. R. Vande Castle, C. H. Calisher, S. T. Nichol, K. D. Abbott, J. C. Young, M. L. Morrison, B. J. Beaty, J. L. Dunnum, R. J. Baker, J. Salazar-Bravo, and C. J. Peters. 2002 The ecology and evolutionary history of an emergent disease: Hantavirus pulmonary syndrome. Bioscience 52:989-998.
Zar, H. Z. 1996. Biostatistical Analysis. Prentice Hall
1Current address: Department of Microbiology, Immunology and Pathology, Colorado State University, 1619 Campus Delivery, CO 80523
Received 16 November 2010
Accepted 29 June 2011